Ultrafast amplifiers are pivotal in enhancing the capabilities of ultrafast laser technology across various domains. These devices scale up the power of laser pulses, enabling groundbreaking applications in fields such as scientific research, material processing, and biomedical imaging. In this article, we look into the mechanics of ultrafast amplifiers, compare them with non-amplified systems, and explore their diverse applications. Here is a quick overview of the topics we cover in this blog post:
Table of Contents:
- Introduction
- What Are Ultrafast Amplifiers?
- Amplified vs. Non-Amplified Systems
- Revolutionary Applications of Ultrafast Amplifiers
- Case Studies and Real-World Impact
- Challenges and Future Directions
- Conclusion
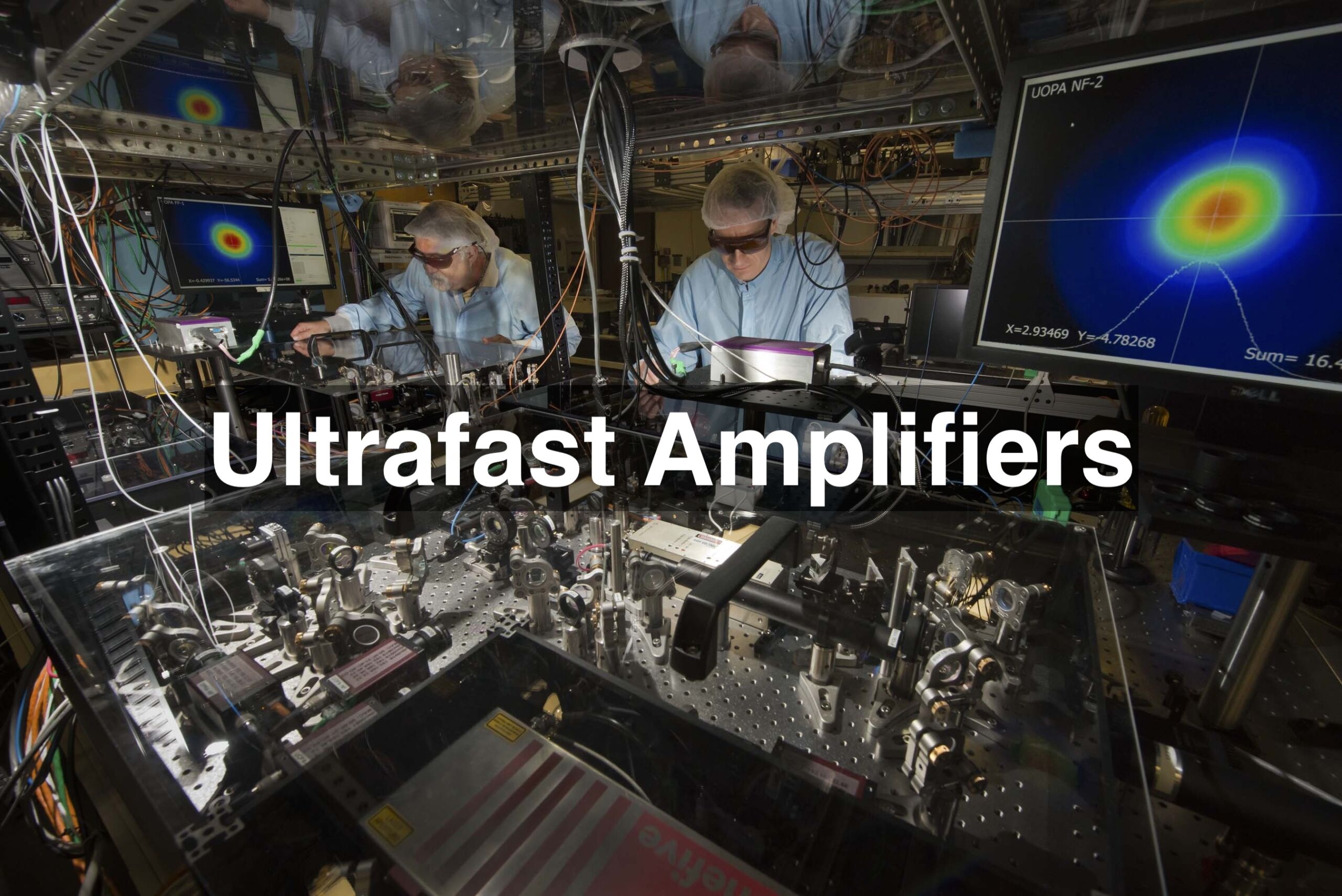
Inside the Lab: Scientists fine-tune ultrafast amplifier systems to push the boundaries of science and technology. Image courtesy of University of Rochester, Laboratory for Laser Energetics
This blog post is brought to you by LECC Technology - Pioneering Laser Technologies for Global Innovation for Over 20 Years
1. Introduction
Ultrafast amplifiers are specialized optical amplifiers that significantly enhance ultrashort laser pulses, typically in the femtosecond (fs) or picosecond (ps) range. These devices can amplify pulses from a low energy level to a much higher one, often with enormous gains, allowing for high pulse energies and correspondingly vast peak powers. The operational principle behind these amplifiers involves taking a train of fast, ultrafast pulses from a seed laser—such as a mode-locked laser—and amplifying them to produce higher average power and pulse energy. This process can achieve optical intensities sometimes exceeding 10^16 W/cm^2, essential for cutting-edge applications in scientific research and industry.
The importance of ultrafast amplifiers lies in their ability to provide the high peak powers necessary for studying and manipulating matter on a very short time scale. This capability is pivotal for scientific research fields, enabling detailed observation and control over fast physical, chemical, and biological processes that are otherwise inaccessible with non-amplified systems. Scientists rely on these powerful pulses for high-precision applications such as particle acceleration, material processing, and generating high harmonics, which are crucial for exploring new aspects of photonics and quantum dynamics.
By bridging the gap between the fundamental generation of ultrashort pulses and their practical use at high energies, ultrafast amplifiers open up a myriad of possibilities for advancing technology and expanding our understanding of the microscopic world.
2. What Are Ultrafast Amplifiers?
Ultrafast amplifiers are advanced devices that increase the intensity and energy of ultrashort laser pulses, which typically last only a few femtoseconds (fs) to picoseconds (ps). These amplifiers are crucial in applications requiring extremely high peak powers that the initial laser systems, often referred to as seed lasers, cannot provide alone.
Function and Technology
The core function of an ultrafast amplifier is to take the low-energy pulses generated by a seed laser and boost their power to much higher levels. This process involves several stages, starting with the initial pulse generation from the seed laser, which could be a mode-locked laser or a gain-switched laser diode. Once these pulses are generated, they enter the amplification stages, which can include:
- Pre-amplification: This stage uses either fiber amplifiers for moderate power levels or bulk regenerative amplifiers to prepare the pulses for further amplification.
- Power Amplification: This can be achieved through various configurations:
- Single-pass Amplifiers: These amplify the pulse as it passes once through the amplifier medium.
- Multipass Amplifiers: Here, pulses circulate multiple times through the amplifier medium to incrementally increase their energy.
- Regenerative Amplifiers: These use a combination of mirrors and a gain medium to repeatedly pass the pulse through the amplifier until it reaches the desired energy level.
- Chirped Pulse Amplification (CPA): This technique is particularly important for maintaining pulse quality at high energy levels. Pulses are first stretched in time to reduce their peak power, amplified, and then recompressed back to their original duration. This process helps avoid damage to the amplifier material and maintains the integrity of the pulse.
Scaling Up Power and Intensifying Laser Pulses
Ultrafast amplifiers intensify pulses by dramatically increasing both their energy and peak power. This amplification is measured in decibels (dB) and can vary significantly based on the amplifier design and the application requirements. For example, some systems may only require a 20 dB increase to achieve the desired power, while others might need over 70 dB for applications demanding extremely high powers.
The capability to scale up power efficiently is due to several factors:
- High Gain: Many ultrafast amplifiers are capable of very high gains, necessary for applications requiring intense bursts of energy over very short times.
- Energy Storage: Unlike continuous-wave lasers, ultrafast amplifiers capitalize on the ability to store energy in the gain medium, which can then be released in powerful bursts. This is critical for maintaining high peak powers without the need for equally high continuous pump power.
- Pulse Picking: In some setups, a device called a pulse picker is used to reduce the repetition rate of the pulses entering the amplifier, allowing for higher energy accumulation per pulse.
Ultrafast amplifiers are indispensable tools in modern photonics labs and are key to pushing the boundaries of what’s possible in both fundamental research and practical applications like materials processing and medical imaging. Their ability to deliver high power in precisely controlled bursts makes them an important technology in advancing our understanding and manipulation of the natural world.
3. Amplified vs. Non-Amplified Systems
Before we move further, let’s clarify what constitutes amplified (e.g., ultrafast amplifiers) and non-amplified systems (e.g., ultrafast oscillators).
Amplified Systems: Amplified systems, such as ultrafast amplifiers, are designed to significantly increase the power, energy, and intensity of ultrashort laser pulses generated by a seed source. These systems take the initial low-energy pulses from the seed laser and enhance them through various amplification stages, which can include techniques like chirped pulse amplification (CPA) or regenerative amplification.
Non-Amplified Systems: Non-amplified systems typically refer to the seed lasers themselves, which include ultrafast oscillators. These devices generate the initial ultrashort pulses that are used in amplified systems. Ultrafast oscillators, such as mode-locked lasers, can produce pulses with durations in the femtosecond range but usually at lower energy levels and peak powers compared to amplified outputs.
Enhancement of Power and Intensity
Ultrafast amplifiers enhance the power and intensity of laser pulses by employing high-gain mediums and techniques that allow the pulses to accumulate energy over multiple passes. This process not only increases the pulse’s peak power but also maintains, to a large degree, the pulse duration, effectively intensifying the pulse without altering its fundamental characteristics. In contrast, non-amplified systems may generate pulses with sufficient speed and precision for some applications but lack the power necessary for processes requiring high energy inputs, such as deep material penetration or high harmonic generation.
Performance Comparison
Efficiency and Output Power: The primary performance benefits of using ultrafast amplifiers over non-amplified systems lie in their vastly superior efficiency and output power. Amplified systems can convert a significant portion of the input energy into usable laser light at very high intensities, which is crucial for applications that depend on strong, focused energy bursts.
- Higher Peak Powers: Amplified systems can achieve peak powers that are several orders of magnitude higher than those possible with non-amplified systems. For instance, while a typical ultrafast oscillator might deliver peak powers in the range of kilowatts to megawatts, an amplified system can boost this to gigawatts or even terawatts.
- Greater Pulse Energy: Amplifiers can increase the energy per pulse from nanojoules (nJ) or microjoules (µJ) typically emitted by oscillators to millijoules (mJ) or even joules (J) per pulse. This increase is crucial for applications requiring high energy density, such as precision micromachining or particle acceleration.
- Application-Specific Benefits: In material processing, higher pulse energies allow for deeper and cleaner cuts or ablations. In medical applications like phototherapy or surgical ablation, amplified pulses ensure effective treatment depths while minimizing thermal damage to surrounding tissues. In scientific research, the high peak powers enable the exploration of nonlinear optical phenomena and the probing of matter on atomic and electronic timescales.
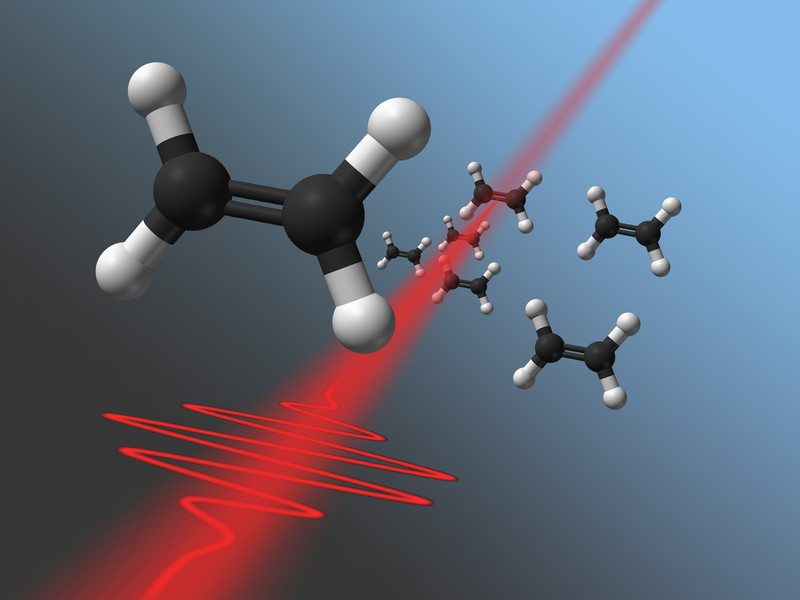
Ultrafast amplifiers can help steer chemical reactions with more powerful pulses.
In summary, while ultrafast oscillators are indispensable for generating the initial pulses, ultrafast amplifiers are essential for elevating these pulses to the power and energy levels required for advanced technological and scientific applications. The choice between amplified and non-amplified systems ultimately depends on the specific needs of the application, considering factors like precision, intensity, and throughput.
4. Revolutionary Applications of Ultrafast Amplifiers
4.1. Fundamental Physics and Chemistry
Since the advent of the first ultrafast laser, substantial progress has been made in understanding the causal relationships of numerous physical and chemical phenomena. Despite this, many details about the transitional stages of these processes remained elusive until the development of ultrafast amplifiers. These devices have enabled researchers to probe incredibly fast femtosecond to picosecond reactions such as molecular vibrations, charge transfers, molecular conformation changes, and electron-hole scattering with unprecedented detail.
Femtochemistry stands out as a field profoundly impacted by ultrafast amplification. This discipline provides insights into the dynamics of chemical reactions, offering clarity on why certain reactions pursue specific pathways. The technique commonly employed here is pump-probe spectroscopy, where a reaction is initiated by a pumping pulse and its progression is monitored through subsequent probing pulses. This method has led to significant discoveries, including the dynamics of stem-loop RNA structures.
Moreover, ultrafast amplifiers have expanded the scope of high harmonic generation, enabling access to the attosecond scale. Systems equipped with Ti-Sapphire lasers and regenerative amplifiers isolate short laser pulses to probe even shorter processes, like charge transfers in photosynthetic reaction centers and the excited states of DNA. These advancements could lead to more efficient solar energy collection and new insights into diseases like skin cancer.
4.2. Material Processing
Ultrafast amplifiers have also transformed the material processing industry. Techniques like photolithography and micro-machining benefit significantly from the precision and power provided by amplified femtosecond pulses. These pulses are capable of creating intricate nanoscale patterns on photoresists and machining materials with minimal heat damage due to their extremely short interaction time, which prevents heat diffusion and electron-phonon coupling from affecting surrounding areas.
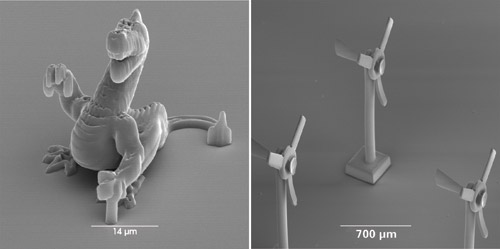
Fine microstructures made by femtosecond laser micromachining.
A notable application is in the safe cutting of explosives, where femtosecond laser pulses minimize thermal transfer and shock waves, allowing for the material to be ablated before any hazardous chemical reactions occur. Furthermore, the ability to deliver laser energy via nonlinear optical absorption enables the creation of features smaller than the diffraction limit, leading to the production of three-dimensional submicron structures. These advancements are not only pivotal in fields like optical communications and data storage but have also made commercial applications like femtosecond LASIK eye surgery possible.
4.3. Bio-Imaging with Ultrafast Amplifiers
In recent years, the capabilities of ultrafast amplifiers have been increasingly harnessed for bio-imaging applications. These systems are particularly advantageous for constructing 3D images of thick specimens, where they outperform traditional fluorescence microscopes by eliminating blurring caused by out-of-focus signals through two-photon excitation of fluorescent dyes. The resultant images offer higher brightness and contrast while minimizing potential damage to living tissues due to controlled power outputs.
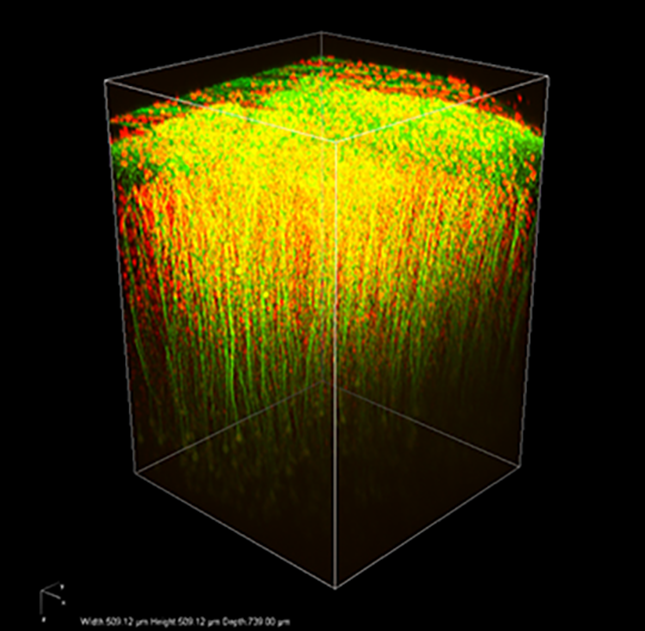
A multicolor image of live mouse brain, made by ultrafast laser systems. Courtesy of Naoki Honkura and Takeshi Imamura
Additionally, ultrafast amplifiers have facilitated the development of terahertz pulse imaging (TPI), a groundbreaking diagnostic tool for epithelial cancer. Unlike traditional methods that rely on visual inspection followed by biopsy, TPI can non-invasively differentiate between cancerous and healthy tissues, potentially eliminating the need for invasive diagnostic procedures.
4.4. Nuclear “Alchemy”
Perhaps one of the most dramatic demonstrations of the power of ultrafast amplifiers is their application in what might be termed “nuclear alchemy.” At Lawrence Livermore National Laboratory in 1999, researchers used a 1.5 petawatt laser pulse lasting 0.5 femtoseconds to induce the transmutation of gold into platinum. The laser pulses were so strong that one out of very 10 billion of gold nuclei was induced to decay to platinum. This marked the first nuclear reaction driven solely by laser light, showcasing the extraordinary potential of ultra-high power lasers to alter the very building blocks of matter.
5. Case Studies and Real-World Impact
Ultrafast amplifiers have not only revolutionized theoretical research but have also been integral to tangible technological advances and medical breakthroughs. In this section, we look into some specific case studies to highlight how these devices can perform effectively in real-world scenarios.
5.1. Femtosecond LASIK Eye Surgery with Ultrafast Amplifiers
One of the most impactful applications of ultrafast amplifiers is in the field of ophthalmology, particularly in LASIK eye surgery. Traditionally, LASIK was performed using mechanical microkeratomes to create a flap in the cornea, which could lead to various complications and inconsistencies in outcomes. The advent of femtosecond lasers, amplified to the necessary energy levels by ultrafast amplifiers, allowed for the creation of the corneal flap with laser precision. This method significantly enhances the accuracy and safety of the procedure.
The use of amplified femtosecond pulses offers a number of advantages:
- Precision: The laser can create extremely fine and consistent cuts, which is crucial for the delicate work required on the corneal tissue.
- Safety: Reduced risk of complications associated with mechanical cutting, such as uneven flap edges or free caps.
- Recovery: Patients generally experience quicker and less painful recovery due to the minimally invasive nature of the laser procedure.
This advancement has not only improved patient outcomes but has also expanded the accessibility of LASIK to a broader population, solidifying its status as a preferred method for correcting vision impairments.
5.2. RNA Structural Dynamics using Ultrafast Amplifiers
In the realm of biochemistry, ultrafast amplifiers have enabled significant advances in understanding RNA structural dynamics, particularly through studies involving femtochemistry techniques like pump-probe spectroscopy. One notable study revealed the dynamics of stem-loop RNA structures, which are crucial for various biological processes, including the regulation of gene expression.
The amplified femtosecond pulses allow researchers to:
- Observe Real-Time Changes: Track and visualize how RNA molecules fold and unfold in real time, which is essential for understanding their function at a molecular level.
- Identify Reaction Pathways: Determine the pathways and intermediate states that RNA molecules undergo during folding, offering insights into how genetic information is regulated.
These insights are vital for developing new therapeutic strategies, particularly in targeting diseases at the genetic level, and could lead to advancements in gene therapy and molecular medicine.
5.3. High Harmonic Generation for Attosecond Pulse Production
High harmonic generation (HHG) is a process where the pulses from ultrafast amplifiers generate high-frequency light from lower-frequency light. This technique has been pivotal in producing attosecond pulses, which are one of the shortest pulses of light ever created, and are used to study electron dynamics in atoms and molecules.
Case Study:
- Experimental Setup: Researchers use an amplified femtosecond laser to ionize a gas, leading to the emission of harmonics that are much higher in frequency than the original light.
- Applications: These attosecond pulses are crucial for time-resolved spectroscopy, allowing scientists to observe electron movements within atoms, thereby opening up new areas of quantum dynamics.
The ability to generate and control such short pulses has fundamentally changed our approach to observing and understanding the quantum world, providing a window into previously unobservable phenomena.
6. Challenges and Future Directions for Ultrafast Amplifiers
Ultrafast amplifiers, while transformative, face significant challenges such as managing high optical intensities which can lead to nonlinear effects and potential damage to the amplification medium. The complexity and cost of these systems also limit their widespread adoption, particularly in industrial applications where robustness and ease of use are paramount.
Current research focuses on enhancing the robustness and decreasing the cost of ultrafast amplifier systems. Innovations include the development of more durable materials that can withstand intense laser pulses without degrading. There is also a significant push to refine chirped pulse amplification and divided-pulse amplification techniques to manage and distribute the energy of amplified pulses more efficiently, minimizing damage while maximizing output.
Looking ahead, the evolution of ultrafast amplifiers is ready to impact numerous fields profoundly. In medicine, advancements could lead to more precise and less invasive surgical techniques. In manufacturing, enhanced ultrafast amplifiers may enable the production of complex materials and devices at the nanoscale, which could revolutionize electronics and materials science. Furthermore, continued improvements in pulse control and energy efficiency could unlock new research opportunities in physics and chemistry, potentially leading to discoveries about the fundamental properties of matter.
By addressing these challenges, the next generation of ultrafast amplifiers will not only become more accessible but also expand their utility across scientific research, healthcare, and industry, signaling an era of unprecedented technological capabilities.
7. Conclusion
Ultrafast amplifiers have by now extended the boundaries of both science and technology, enabling deeper insights and more precise interventions across a spectrum of disciplines. From revolutionizing surgical procedures with femtosecond LASIK to unveiling the dynamics of molecular and atomic interactions, these devices have opened new vistas in understanding and manipulating the natural world.
The versatility and impact of ultrafast amplifiers suggest that their future applications will be as transformative as those they currently enable. As the technology continues to evolve, its integration into new areas of research and industry promises to bring about further groundbreaking developments.
For professionals looking to explore the technical specifics and potential applications of these powerful tools, a wealth of information is available. To learn more about how ultrafast amplifiers can enhance your research or industrial capabilities, visit FindLight’s comprehensive ultrafast laser page.
This journey into the capabilities of ultrafast amplifiers shows that we are only at the beginning of discovering what these tools can achieve. The continued refinement and application of ultrafast amplifiers will undoubtedly pave the way for future innovations that we can scarcely imagine today.
[…] ← Previous […]