Achieving ultrafast laser pulses with high energy and precision is key to breakthroughs across various research fields. From micromachining advanced materials to driving innovations in biomedical imaging and spectroscopy, the demand for intense, short-duration laser pulses is growing rapidly. However, generating such powerful pulses without compromising their speed or quality is a challenge — one that multipass ultrafast amplifiers are designed to overcome. These amplifiers amplify seed pulses to incredibly high energies while preserving their ultrafast characteristics, making them indispensable tools in both research labs and industrial settings. In this guide, we’ll explore the fundamentals of multipass amplification, unpack its inner workings, and examine why it is central to advancing today’s ultrafast laser technology.
This guide is brought to you by RPMC Lasers - a Leading American Supplier of laser equipment.
1. Introduction to Multipass Ultrafast Amplifiers
Multipass ultrafast amplifiers are specialized solid state lasers designed to enhance the energy of short laser pulses, typically in the femtosecond to picosecond range, by guiding them through a gain medium multiple times. Unlike regenerative amplifiers, which rely on a cavity to circulate pulses and achieve amplification, multipass amplifiers circulate the pulse externally through a series of optics without being trapped in a cavity. This design not only allows for significant energy amplification but also reduces the risks of pulse broadening, making it possible to achieve shorter pulse durations and higher peak powers.
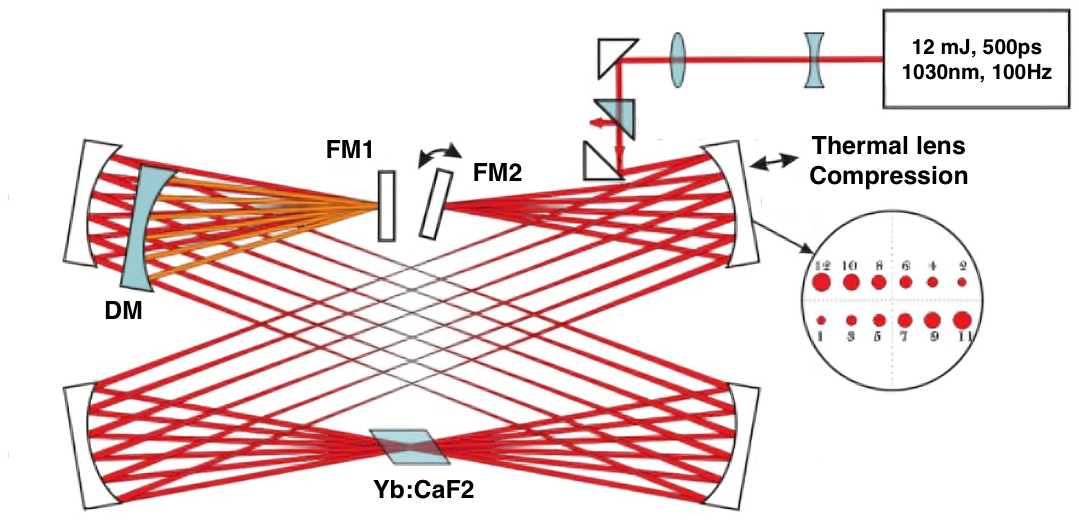
Diagram showing the configuration of a typical multipass ultrafast amplifier, highlighting the flow of seed pulses, gain medium, and amplification process. Image courtesy of Kaksis, Edgar & Almasi, Gabor & Fülöp, József & Pugžlys, A. & Baltuška, A. & Andriukaitis, Giedrius. (2016). 110-mJ 225-fs cryogenically cooled Yb:CaF2 multipass amplifier. Optics Express. 24. 28915. 10.1364/OE.24.028915.
The importance of multipass ultrafast amplifiers spans various high-impact applications. In material processing, their high peak powers enable precise micromachining, minimizing thermal damage while cutting or structuring materials. In spectroscopy, they generate the intense, coherent light sources needed for nonlinear and time-resolved studies, while in biomedical imaging, they facilitate techniques like multiphoton microscopy, allowing deep tissue penetration with minimal photodamage.
Given the growing demand for high-energy, ultrafast pulses in both research and industrial contexts, multipass amplifiers stand out for their ability to deliver shorter, more intense pulses than other amplification techniques. For engineers and scientists engaged in laser development, understanding the design, functionality, and performance advantages of multipass amplifiers is crucial for optimizing laser setups or advancing new applications. This guide delves into their working principles, components, and key factors governing performance, offering a good foundation for understanding ultrafast laser systems.
2. Fundamentals of Ultrafast Amplification
Ultrafast laser pulses are defined by their extremely short durations, typically ranging from femtoseconds (10⁻¹⁵ seconds) to picoseconds (10⁻¹² seconds). These pulses pack significant energy into a narrow temporal window, enabling high peak powers that are crucial for a range of scientific, industrial, and biomedical applications. The ability to deliver such rapid bursts of light is what makes ultrafast lasers indispensable in fields like spectroscopy, micromachining, and multiphoton imaging, where precision and minimal thermal impact are required.
2.1. Why Amplification is Necessary
While ultrafast lasers generate short-duration pulses with high peak power, their initial pulse energy — often produced by an ultrafast oscillator — tends to be relatively low, usually in the nanojoule to microjoule range. This energy is insufficient for many applications that require higher intensities to induce nonlinear effects, process materials, or penetrate deeper into biological tissues.
Amplification is thus necessary to increase the pulse energy to levels that enable more demanding applications. The challenge, however, lies in achieving this energy boost without compromising the pulse’s short duration or causing pulse distortion. Conventional amplification methods can introduce pulse broadening and distortion due to dispersion and nonlinear effects, making it difficult to maintain the original ultrafast characteristics.
2.2. How Multipass Amplifiers Achieve High Peak Power
Multipass amplifiers are designed specifically to increase pulse energy while preserving the ultrafast nature of the pulse. Unlike single-pass or regenerative amplifiers, multipass amplifiers circulate the pulse multiple times through a gain medium, incrementally boosting its energy with each pass.
Key aspects that make multipass amplification effective in achieving high peak powers without pulse distortion include:
- Multiple Circulations: By allowing the pulse to pass through the gain medium several times, multipass amplifiers achieve higher gain without a drastic increase in medium thickness. This minimizes dispersion and nonlinear effects, maintaining the integrity of the pulse’s short duration.
- Thinner Gain Media: As discussed earlier, thinner gain media contribute less dispersion, which helps preserve the temporal profile of the pulse. The pulse retains its initial shape more effectively, reducing the need for complex post-amplification compression.
- Flexible Design: Multipass amplifiers offer flexible configurations that allow researchers to optimize the number of passes, pulse alignment, and gain medium type, making them versatile tools in a variety of experimental setups.
Multipass ultrafast amplification thus stands out as a critical technique for maintaining both high pulse energy and short durations. By balancing gain with minimal distortion, these amplifiers enable precise, high-intensity applications that leverage the full potential of ultrafast laser technology.
3. Principle of Operation
Multipass amplifiers are designed to amplify ultrafast laser pulses by circulating them through a gain medium multiple times. This approach incrementally boosts pulse energy while minimizing temporal broadening and other distortions. Let’s break down the operational details.
3.1. Seed Pulses: Starting Point and Characteristics
The process begins with a seed pulse generated by an ultrafast laser oscillator. These seed pulses typically have low energy (in the nanojoule to microjoule range) but exhibit very short durations, usually in the femtosecond to picosecond range. The goal of the multipass amplifier is to increase the energy of these pulses while preserving their ultrashort characteristics.
3.2. Multipass Architecture: Circulating Pulses through the Gain Medium
The core of the multipass amplifier is its architecture, which consists of a series of optics — mirrors and lenses — that guide the seed pulse repeatedly through the gain medium. Unlike single-pass amplifiers, where the pulse passes through the gain medium only once, multipass amplifiers use carefully aligned optical elements to circulate the pulse multiple times, optimizing energy extraction from the medium.
Each pass through the gain medium amplifies the pulse incrementally. The optics ensure that the pulse retraces its path with minimal losses and maintains its spatial profile throughout the amplification process. The design allows for flexible adjustment of the number of passes, which can be tailored based on the desired output energy and the characteristics of the gain medium.
3.3. Role of the Gain Medium
The gain medium is central to the amplification process, providing the energy needed to boost the pulse. Common gain media include:
- Ti:sapphire: Offers a broad gain bandwidth, making it suitable for amplifying femtosecond pulses across a wide range of wavelengths.
- Yb-doped crystals (e.g., Yb, Yb₂): Known for their efficiency, these materials are ideal for picosecond amplification, with lower thermal effects and better energy efficiency.
The gain medium absorbs pump energy (typically from a continuous or pulsed laser) and transfers it to the seed pulse through stimulated emission. Thinner gain media are often preferred to minimize dispersion and pulse broadening, ensuring that the pulse duration remains as short as possible throughout the amplification process.
3.4. Energy Increase with Each Pass
As the seed pulse circulates through the gain medium, it extracts more energy with each pass. The total gain is the product of the individual gains from each pass. By carefully controlling the number of passes, multipass amplifiers can achieve high pulse energies — often in the millijoule to joule range — while maintaining the pulse’s ultrafast duration and spatial quality.
The ability to control the number of passes also allows for optimization based on specific requirements, such as minimizing gain saturation (where the medium’s amplification capacity becomes depleted) or managing nonlinear effects like self-phase modulation.
3.5. Comparison with Other Amplification Methods
Let’s look briefly at a few of the common amplification architectures to understand pros and cons of each:
Single-Pass Amplifiers:
- These amplifiers direct the pulse through the gain medium once, resulting in lower overall gain. While simpler in design, single-pass amplifiers cannot achieve the same energy levels as multipass amplifiers without introducing thicker gain media, which increases dispersion and pulse distortion.
- Advantage: Simplicity and lower cost.
- Challenge: Limited gain and higher risk of pulse broadening.
Regenerative Amplifiers:
- In regenerative amplification, the seed pulse is trapped inside a cavity, circulating many times until it reaches the desired energy level. The cavity architecture introduces more potential for pulse distortion and can complicate pulse extraction timing.
- Advantage: High gain and precise pulse control. Offers a straightforward design and relatively easy alignment compared with its multipass counterpart.
- Challenge: Pulse duration is often longer due to nonlinear effects (longer gain medium), and the complexity of timing synchronization makes it less suitable for extremely short pulses.
Multipass Amplifiers:
- Multipass amplifiers allow for efficient amplification without confining the pulse in a cavity, reducing the potential for pulse distortion and timing issues. By enabling the pulse to make multiple external passes through the gain medium, they can achieve high peak power while preserving the ultrafast nature of the pulse.
- Advantage: High energy, shorter pulse duration, reduced complexity in timing, and minimal distortion.
- Challenge: Requires precise alignment and a well-calibrated optical system to ensure consistent energy gain and pulse quality.
Multipass amplifiers thus offer a balanced solution for achieving high peak power without compromising pulse duration, making them the preferred choice in many advanced laser applications.
4. Key Components of Multipass Amplifiers
Multipass ultrafast amplifiers rely on a combination of precise components to effectively amplify short-duration pulses while maintaining high energy and peak power. Below are the critical components and their roles in the amplification process.
4.1. Seed Source
The seed source for a multipass amplifier is typically a laser oscillator that generates initial ultrafast pulses. Key characteristics of the seed pulses include:
- Short pulse duration: Femtosecond to picosecond range, depending on the application.
- Low initial energy: Usually in the nanojoule to microjoule range.
- High temporal coherence: Essential for maintaining phase integrity during amplification.
Seeding requirements depend on the gain medium and the intended energy output. For effective amplification, the seed pulses must have adequate spectral overlap with the gain bandwidth of the medium, ensuring efficient energy transfer with minimal spectral or temporal distortion.
4.2. Gain Medium
The gain medium is the core component that provides energy to the pulses. The choice of material and its doping levels determine the amplification characteristics:
- Material types: The most common gain media in multipass amplifiers are Ti- and Yb-doped crystals, each suited for specific pulse durations and wavelength ranges.
- Ti:sapphire: Offers a broad gain bandwidth, making it suitable for femtosecond pulse amplification over a wide wavelength range (typically 700-1100 nm).
- Yb-doped crystals (e.g., Yb, Yb₂): Offer high efficiency for picosecond amplification, with narrower gain bandwidths and lower thermal effects.
- Doping levels: The concentration of the dopant in the crystal impacts the gain coefficient and saturation energy. Higher doping levels increase gain but may also introduce nonlinear effects and thermal challenges.
- Energy transfer efficiency: This refers to how well the gain medium converts pump energy into pulse energy. Efficient gain media reduce energy losses and minimize thermal loading, which is crucial for maintaining consistent pulse amplification.
4.3. Optics
Multipass amplifiers require a carefully designed optical setup to ensure that pulses make multiple passes through the gain medium:
- Mirrors: High-reflectivity broadband mirrors are used to redirect the pulses, ensuring minimal energy loss at each reflection. The alignment of these mirrors is critical for maintaining the spatial profile and temporal characteristics of the pulse.
- Lenses: Focusing lenses or telescopic lens systems help control the beam’s focus and divergence, optimizing the interaction between the pulse and the gain medium.
- Beam shaping elements: These elements are used to maintain beam quality and ensure uniform energy distribution across the pulse profile. Examples include spatial filters, apertures, and beam expanders.
The optics must be precisely aligned to achieve consistent energy gain with each pass, minimize losses, and prevent any undesired pulse distortion.
4.4. Chirped Pulse Amplification (CPA)
Chirped Pulse Amplification (CPA) is often integrated into multipass amplification systems to manage pulse distortions and energy limitations:
- Pulse stretching: Before amplification, the seed pulse is stretched in time using a pair of gratings or a dispersive stretcher. This reduces the peak intensity and prevents damage to the gain medium and optics during amplification.
- Pulse amplification: Once stretched, the pulse is amplified through multiple passes in the gain medium.
- Pulse compression: After amplification, the pulse is recompressed to its original ultrashort duration using a pair of gratings or a prism compressor, restoring its high peak power.
CPA is essential when dealing with extremely short femtosecond pulses, as it mitigates nonlinear effects like self-phase modulation and ensures safe amplification.
4.5. Cooling System
Thermal management is critical in multipass amplifiers, as the gain medium and optics can generate significant heat during operation:
- Heat dissipation: Effective cooling prevents thermal lensing, which can distort the beam’s spatial profile and reduce amplification efficiency.
- Cooling methods: Water cooling, thermoelectric cooling, or even cryogenic cooling can be employed depending on the power level and type of gain medium.
- Thermal stability: Consistent cooling ensures stable performance and prevents variations in pulse energy and duration, maintaining the amplifier’s reliability and longevity.
By integrating these components effectively, multipass amplifiers can achieve high-energy pulses with minimal distortion, enabling a wide range of scientific and industrial applications.
5. Design Considerations
Designing a multipass ultrafast amplifier requires careful optimization to achieve high energy output while preserving pulse quality and duration. Several critical factors influence the performance, efficiency, and stability of the amplification process.
5.1. Number of Passes
The number of passes through the gain medium is a key factor in determining the amplifier’s overall gain:
- Optimal Number: The ideal number of passes is a balance between achieving the desired energy gain and maintaining beam quality. More passes increase the energy extracted from the gain medium but also introduce greater risks of dispersion and nonlinear effects.
- High gain requirement: If the goal is maximum pulse energy, the number of passes is increased until gain saturation is approached. However, beyond a certain point, additional passes yield diminishing returns due to the depletion of the gain medium’s inversion and increased potential for pulse distortions.
- Beam quality: If high beam quality is critical, a moderate number of passes may be favored to minimize the cumulative effects of dispersion and self-phase modulation. For example, a 4-8 pass setup is common in femtosecond amplifiers to achieve a balance between gain and beam preservation.
5.2. Beam Quality
Maintaining high-quality beam profiles throughout the amplification process is essential for applications that require precise beam focus or delivery:
- Strategies for maintaining beam quality:
- Beam shaping: Using adaptive optics or spatial filters can help correct distortions and maintain a uniform energy distribution across the pulse profile.
- Minimizing aberrations: High-quality optics (e.g., low-aberration mirrors and lenses) and precise alignment can reduce the introduction of beam distortions during each pass.
- Managing thermal effects: As the gain medium heats up, it can cause thermal lensing, which alters the beam’s spatial characteristics. Efficient cooling systems help maintain consistent beam quality by reducing thermal gradients within the gain medium.
5.3. Pulse Distortion
Pulse distortion is a significant concern in ultrafast amplification, as even small distortions can affect pulse duration, shape, and coherence:
- Common sources of distortion:
- Dispersion: Chromatic dispersion occurs as different wavelengths travel at different speeds through the gain medium and optical elements. This effect spreads the pulse temporally, reducing peak intensity.
- Nonlinearities: Nonlinear effects, such as self-phase modulation and Kerr lensing, can occur at high peak intensities, altering the phase and shape of the pulse.
- Minimizing pulse distortion:
- Using thinner gain media: Thinner gain media reduce the amount of material-induced dispersion and nonlinearity, preserving the temporal profile of the pulse.
- Incorporating CPA: Stretching the pulse before amplification reduces peak intensity, lowering the impact of nonlinearities and enabling safer amplification.
- Precise optical design: Optimizing the optical path for minimal dispersion and reflection-induced phase shifts is essential for maintaining pulse coherence and short durations.
5.4. Alignment
Precise optical alignment is critical for consistent and efficient amplification in multipass amplifiers:
- Importance of alignment: Inaccurate alignment can lead to losses, beam divergence, and pulse distortions, affecting overall gain and output quality. Even small misalignments can cause energy leakage, reduce amplification efficiency, and degrade beam quality.
- Techniques for achieving precision:
- Active feedback systems: Using automated feedback systems, such as beam position sensors and actuators, helps maintain optimal alignment over time, compensating for thermal shifts and mechanical drifts.
- Manual fine-tuning: Initial setup often involves manual adjustments with high-precision mounts and translation stages to ensure that the pulse retraces its path accurately through each pass.
- Stable optical mounts: High-stability mounts and vibration isolation platforms minimize the impact of mechanical vibrations, ensuring consistent pulse amplification.
By carefully managing these design considerations, multipass amplifiers can achieve high peak powers, maintain beam quality, and preserve ultrashort pulse durations, making them well-suited for demanding scientific and industrial applications.
6. Applications of Multipass Ultrafast Amplifiers
Multipass ultrafast amplifiers are instrumental in a variety of high-precision applications, enabling processes that require both high peak power and ultrashort pulse durations. Below are the key use cases where these amplifiers make a significant impact.
6.1. Material Processing
Multipass ultrafast amplifiers are widely used in industrial material processing, where their ability to deliver high-intensity, ultrashort pulses is leveraged for:
- Micromachining: Ultrafast pulses can ablate material with extreme precision and minimal heat-affected zones (HAZ). This is ideal for creating intricate features in materials like metals, semiconductors, and polymers. For instance, micromachining of semiconductor wafers for microelectronics manufacturing uses femtosecond laser pulses to create fine structures without causing thermal damage.
- Cutting and Drilling: These amplifiers enable clean, precise cuts, making them suitable for applications like drilling holes in medical stents or cutting brittle materials like glass and ceramics. The short pulse duration ensures that the energy is deposited faster than heat can diffuse, resulting in smooth edges and minimal debris.
- Surface Structuring: By varying pulse parameters, multipass amplifiers can create nanostructures or microstructures on surfaces, altering properties like wettability, reflectivity, or adhesion. This is useful in producing anti-reflective surfaces for optics or creating hydrophobic surfaces in medical implants.
Real-World Example: In the manufacturing of OLED displays, multipass amplifiers are sometimes used for precise micromachining of thin films, enabling the creation of high-resolution screen features.
6.2. Biomedical Imaging
Multipass amplifiers have transformed biomedical imaging, allowing for deep tissue visualization with minimal damage:
- Multiphoton Microscopy: By delivering femtosecond pulses at specific wavelengths, multipass amplifiers enable multiphoton excitation deep within biological tissues. This allows for high-resolution, 3D imaging of living cells, tissues, and even whole organisms, with reduced photodamage compared to continuous-wave lasers.
- Optogenetics: In neuroscience, multipass amplifiers are used to stimulate neurons with high precision. The short pulse duration allows for localized activation of genetically modified cells, enabling detailed studies of neural circuits and behaviors.
Real-World Example: Multipass ultrafast lasers are used in imaging the dynamics of calcium ions in live neurons, providing critical insights into neural signaling and network behavior.
6.3. Spectroscopy
The precise, high-energy pulses generated by multipass amplifiers are essential for various spectroscopy techniques:
- Time-Resolved Spectroscopy: Multipass amplifiers enable the study of ultrafast processes such as chemical reactions, energy transfer in molecules, and phase transitions in materials. By generating femtosecond pulses, they allow researchers to capture dynamic events at the atomic or molecular level, offering insights into reaction kinetics and mechanisms.
- Nonlinear Optics Experiments: The high peak intensities produced by multipass amplifiers facilitate nonlinear optical phenomena, such as harmonic generation, four-wave mixing, and Raman scattering. These processes are critical for studying material properties, generating new wavelengths, or probing molecular structures.
Real-World Example: In the study of photosynthesis, multipass ultrafast amplifiers are used for time-resolved spectroscopy to observe the energy transfer processes within light-harvesting complexes, contributing to understanding and optimizing artificial photosynthetic systems.
Multipass ultrafast amplifiers are fundamental to advancing precision applications in material science, biomedical research, and spectroscopy. Delivering ultrafast, high-energy pulses, they provide the tools necessary for exploring complex phenomena, processing materials, and imaging biological tissues — all with minimal thermal effects and high accuracy.
7. Advancements and Future Trends
Multipass ultrafast amplifiers continue to evolve with advancements in gain media, alignment techniques, and pulse compression methods. These innovations are not only enhancing the performance of current systems but also paving the way for more efficient, stable, and compact designs.
7.1. Recent Innovations in Multipass Amplifiers
- Advanced Gain Media:
- New Yb-doped Crystals: Innovations in Yb-doped gain media, such as Yb and Yb₂, offer broader gain bandwidths, higher efficiency, and better thermal conductivity compared to traditional Yb or Yb₂. This allows for more efficient amplification of ultrashort pulses, enabling higher peak powers with reduced thermal effects.
- Thinner, High-Doping Levels: Researchers are developing thinner gain media with optimized doping levels to enhance energy extraction while minimizing dispersion and nonlinear effects. These materials allow for higher gains per pass, reducing the total number of passes needed and maintaining short pulse durations.
- Ti: Sa Variants: Improvements in Ti: Sa crystals have focused on increasing fluorescence lifetimes and reducing absorption losses. This enhances the energy extraction efficiency and makes Ti: Sa multipass amplifiers more suitable for high-repetition-rate applications.
- Improved Alignment Techniques:
- Automated Beam Alignment Systems: Recent advancements in automated feedback-based beam alignment systems have significantly improved the precision and stability of multipass amplifiers. These systems use sensors and actuators to continuously adjust the optical path, maintaining optimal alignment even during long-duration experiments or under changing thermal conditions.
- Adaptive Optics: Adaptive optics, including deformable mirrors and spatial light modulators, are being integrated into multipass systems to actively correct wavefront distortions. This not only improves beam quality but also ensures consistent energy delivery throughout the amplification process, enhancing system reliability.
- Next-Generation Pulse Compression:
- Grating-Based Compression: Innovations in high-dispersion gratings and compressor design have allowed for more effective recompression of amplified pulses, achieving shorter pulse durations with reduced energy loss.
- Nonlinear Compression Techniques: Emerging nonlinear compression techniques, such as hollow-core fiber-based spectral broadening followed by compression, are pushing the boundaries of pulse durations, achieving sub-10-femtosecond pulses at higher energies. This expands the range of applications in fields like attosecond science and advanced spectroscopy.
7.2. Ongoing Research and Future Trends
Energy Efficiency:
- Researchers are focusing on improving energy conversion efficiency from pump sources to amplified pulses. This involves both optimizing gain medium characteristics and developing more efficient pump lasers, such as diode-pumped solid-state lasers (DPSSLs), which offer higher conversion rates and lower operational costs.
- Enhanced thermal management systems, including more efficient cooling techniques (e.g., cryogenic cooling), are being explored to improve energy efficiency by minimizing heat-induced losses in the gain medium.
Beam Stability:
- Beam stability is critical in applications where consistent intensity and focusability are required. Ongoing research aims to develop more stable optical mounts and vibration-isolation systems to minimize beam deviations during amplification.
- Researchers are also investigating active stabilization systems that can compensate for fluctuations in real time, maintaining beam quality across different environmental conditions and operation times.
Compact Design:
- As demand grows for more portable and integrated laser systems, efforts are underway to reduce the size and complexity of multipass amplifiers. Advances in micro-optics and compact pump sources are enabling the development of smaller, modular amplifier systems without sacrificing performance.
- Integrated systems, which combine seed oscillators, multipass amplifiers, and pulse compression units into a single platform, are emerging as a trend in both research labs and industrial applications. These compact designs offer easier deployment, lower maintenance requirements, and greater versatility across different use cases.
Multipass ultrafast amplifiers are evolving toward higher energy efficiency, improved beam quality, and more compact designs. These trends are driving advancements in precision applications, expanding the capabilities of ultrafast laser technology, and opening new opportunities for scientific discovery and industrial innovation.
8. Conclusion
Multipass ultrafast amplifiers play an important role in advancing laser technology by enabling the amplification of short-duration pulses to high energies while preserving their ultrafast characteristics. From the initial seed pulses to the careful selection of gain media, optics, and alignment, each component and design consideration contributes to achieving the desired balance of energy, beam quality, and minimal pulse distortion. By circulating pulses multiple times through a carefully aligned gain medium, these amplifiers offer a powerful solution that supports a wide range of applications, from precision material processing and biomedical imaging to complex spectroscopy experiments.
The significance of multipass amplifiers lies not only in their ability to deliver high peak powers but also in their contribution to scientific and industrial progress. As new innovations in gain media, alignment technologies, and pulse compression methods continue to emerge, multipass amplifiers will become even more efficient, stable, and compact. This evolution is crucial for both researchers and engineers seeking to push the boundaries of ultrafast laser applications and optimize experimental setups.
For those interested in exploring more about multipass amplifiers, there is a wealth of resources, including research papers, technical manuals, and industry case studies, that delve deeper into specific design aspects and use cases. Engaging in discussions with peers and industry experts can also provide valuable insights and practical knowledge, contributing to the broader field of ultrafast laser technology.
9. Further Reading/References
To explore multipass ultrafast amplifiers in greater depth, here are some key papers, textbooks, and online resources that offer comprehensive insights:
- Textbooks:
- “Ultrashort Laser Pulse Phenomena” by Jean-Claude Diels and Wolfgang Rudolph
This textbook covers the fundamental physics of ultrashort laser pulses, including amplification techniques like multipass amplifiers and CPA systems. It’s an essential reference for both beginners and experienced researchers in ultrafast optics. - “Femtosecond Laser Pulses: Principles and Experiments” by Claude Rullière
This book provides detailed explanations of femtosecond pulse generation, amplification, and applications, with a focus on multipass amplification techniques and nonlinear optical effects.
- “Ultrashort Laser Pulse Phenomena” by Jean-Claude Diels and Wolfgang Rudolph
- Key Papers:
- Backus, S., et al. “High Power Ultrafast Lasers,” Review of Scientific Instruments (1998).
This foundational paper discusses the design principles of high-power ultrafast lasers, including multipass amplification, and outlines the key challenges and solutions in the field. - Brabec, T., Krausz, F. “Intense Few-Cycle Laser Fields: Frontiers of Nonlinear Optics,” Reviews of Modern Physics (2000).
This review covers the generation and amplification of intense ultrashort pulses, detailing the evolution of multipass amplifiers and their role in nonlinear optics.
- Backus, S., et al. “High Power Ultrafast Lasers,” Review of Scientific Instruments (1998).