Combi Probes and how they are employed in multi-modal fiber spectroscopy is the subject of this article.
Fiber optic spectroscopy is a strong analytical technique that uses optical fibers to guide light to and from a sample, enabling real-time, remote, and non-invasive analysis of materials, liquids, and biological tissues. By coupling spectrometers with fiber optics, this method allows for flexible and compact system designs that can operate in harsh or confined environments. It plays a vital role in a wide range of applications. This includes environmental monitoring, biomedical diagnostics, industrial process control, and chemical identification. The ability to perform in situ measurements with high sensitivity and minimal sample preparation makes fiber optic spectroscopy a powerful tool for both research and field-based sensing. Its significance lies in its adaptability, speed, and ability to deliver precise spectral information across various wavelengths, from UV to mid-infrared.
This work is brought to you by art photonics GmbH - leading supplier of specialty fiber products for a broad spectral range from 300nm to 16µm.
1. Technological Foundations of Combi Probes
Dual or multimodal fiber spectroscopy is an emerging technique that integrates two complimentary optical spectroscopies into a single probe system. The goal is to leverage their capabilities while reducing their limitations. Applied separately, single spectroscopic techniques can be restrictive. For instance, Near Infrared spectroscopy (NIR) – a well established technique commonly used in medical diagnosis and pharmaceutical analysis – is well-suited for determining biological molecules and identifying certain functional groups. It is also capable of successfully diagnosing cancer. Since absorption of NIR radiation is typically low in biological samples, this allows the light to propagate deeper into the samples requiring little to no sample preparation. However, due to large absorption bands creating spectral overlap, it is difficult to extract quantitative information using only this spectroscopic method. On the other hand, mid-infrared (MIR) spectroscopy is characterized by sharper absorption peaks, which enhances molecular specificity and improves identification accuracy.
Meanwhile, Raman spectroscopy can be used to identify unknown samples in bulk chemicals and aqueous solutions. By combining these techniques (NIR-Raman or MIR-Raman), Combi Probes overcome the individual limitations of each method and enhance measurement resolutions. This synergy allows users to discriminate overlapping spectral signatures, detect weakly absorbing species, and reduce ambiguity in complex sample which are all essential capabilities in fields such as biomedicine, pharmaceutical QC, and petrochemical monitoring
2. Design and Operation Principles of the Combi Probe
The multimodal operation of spectroscopic optical probes such as Combi Probes, is made possible by the development and integration of new fiber optics designs into a single robust probe. Each spectroscopic technique is characterized by its unique wavelength range: Fluorescence (400 – 700 nm), NIR (700 – 2500 nm), MIR (2500 – 25000 nm).
While silica fiber is excellent for propagating visible and near infrared radiation with little to no loss, its absorption increases significantly for wavelengths greater than 2 um. Therefore, to support wavelengths beyond the Near Infrared Regime, art photonics GmbH employs Chalcogenide Infrared (CIR) and Polycrystalline Infrared (PIR) fibers in the Combi Probes. These fibers are characterized by their high transmission and low absorption for mid infrared wavelengths. By combining silica fibers with infrared fibers, a Combi Probe offers almost full coverage of the optical domain . It thus allows for maximum sample characterization.
Another important design feature of the Combi Probe is the implementation of a carefully engineered tip that allows light collection from a broad spectrum of light.
In addition to selecting a high index material characterized by a large transparency window and low absorption for the wavelength region of interest, ray tracing methods are employed to study the geometry of the tip and ensure high transmittance and low scattering effects which could interfere with data collection. For a Combi Probe that integrates Fluorescence and MIR modalities, Art Photonics uses a zirconium dioxide tip. However, other materials such as diamond are useful for other modalities, although typically increasing the cost.
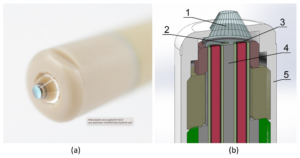
Figure 1. Multispectral Combi Probe head design: (a) photo of the assembled probe; (b) axial cross-section showing key components—1: ATR crystal; 2: silica fibers; 3: CIR fibers; 4: ferrule; 5: probe shaft. Image adapted with permission from Bogomolov et al., Anal. Chem. 2021, 93, 6013–6018. https://doi.org/10.1021/acs.analchem.1c00080. Copyright © 2021 American Chemical Society.
3. Applications of Combi Probes
Application of Combi Probes can play an instrumental role across various industries.
In Process Analytical Technology (PAT), Combi Probes represent a solution for better understanding process, monitoring, and control. The probes can be used as predictive models that detect process deviations.
In-line measurements present another area where Combi Probes can be advantageous. Large manufacturing environments, such as the food and beverage industries, demand reliable and fast evaluation of concentration and chemical composition. Thanks to their ability to make real time and continuous measurements, Combi Probes can ensure consistent product quality all while being operated remotely. For instance, Raman spectroscopy has been extensively used to measure glucose concentrations in chemicals without the need to extract samples. The remote control feature of Combi Probes allows for constant monitoring in production lines without interruption of the manufacturing process.
In the medical field, several recent studies investigated a multimodal spectroscopic approach of scanning to distinguish between cancerous and healthy tissue. It is worth noting that these results make Combi Probes appealing instruments in clinical settings as such tissues can be very complex and difficult to analyze. This is also very important for decision making. It significantly reduces the time it takes to analyze data compared to conventional techniques.
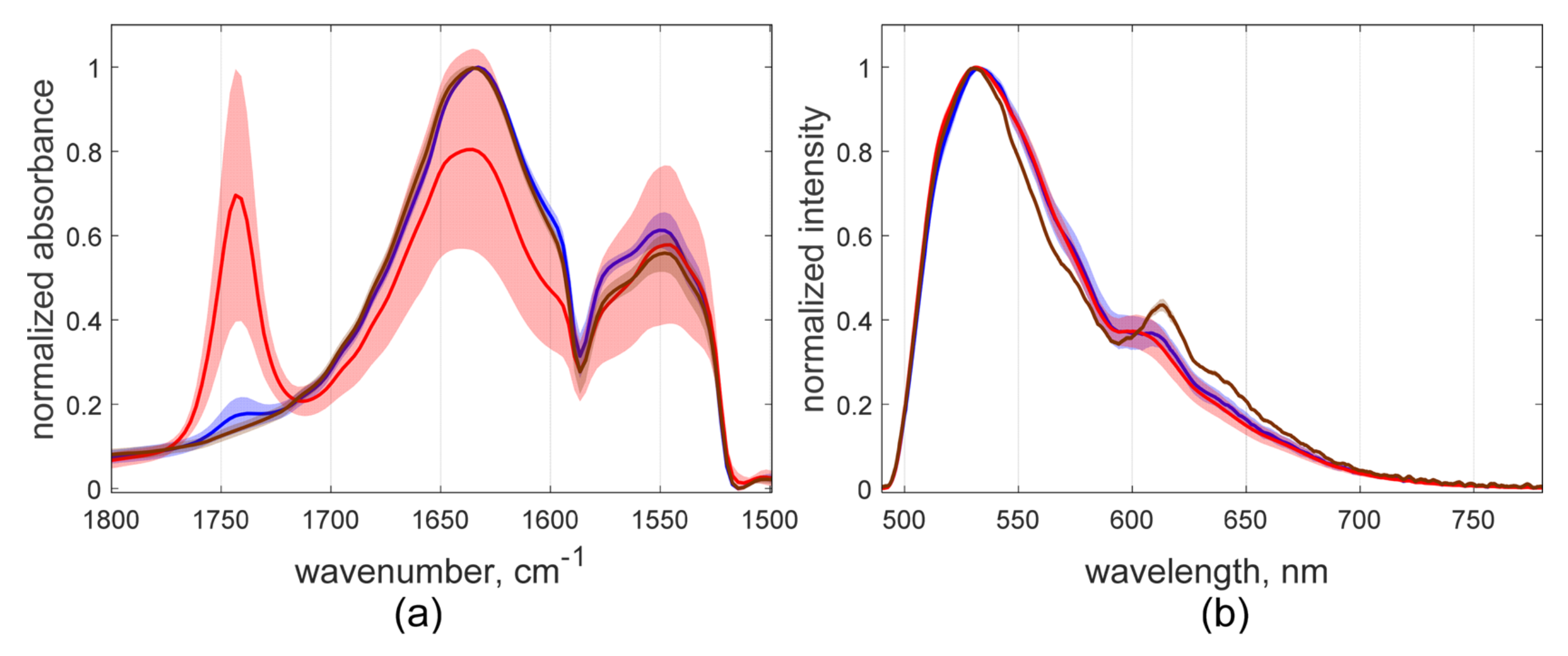
Normalized experimental spectra of biological tissues acquired with the multispectral fiber probe: (a) mid-infrared spectra and (b) fluorescence spectra processed with Standard Normal Variate (SNV). Tissue types include chicken breast (blue), fat (red), and liver (brown). Solid lines represent mean spectral curves, and shaded regions show standard deviation intervals. Image adapted with permission from Bogomolov et al., Anal. Chem. 2021, 93, 6013–6018. https://doi.org/10.1021/acs.analchem.1c00080. Copyright © 2021 American Chemical Society.
4. Advantages of Dual Spectroscopy
Enhanced Data Resolution of Combi Probes
Dual spectroscopy offers a significant improvement in the resolution and reliability of spectral data by integrating complementary modalities that operate in different spectral regimes. For example, Raman spectroscopy detects vibrational modes of molecules with high specificity, providing detailed structural information. However, it suffers from low signal intensity and is susceptible to interference from fluorescence, especially in biological samples.
NIR spectroscopy, on the other hand, measures overtones and combination bands primarily related to C-H, O-H, and N-H vibrations. While it produces broader, less distinct peaks compared to Raman, it is highly effective at quantifying bulk composition and detecting moisture, protein, or fat content.
Comparative studies have shown that single-modality systems often fail to discriminate between chemical bonds. A recent pharmaceutical study showed that NIR spectroscopy coupled with Raman spectroscopy can offer insights on the presence of active pharmaceutical ingredients (API) in drugs.
Comprehensive Data Collection
A key strength of Combi Probes lies in their ability to generate multi-dimensional datasets from a single probe insertion or scan. Each integrated modality contributes a unique layer of information. NIR can quantify water and protein content, MIR characterizes functional groups with high specificity, and fluorescence identifies trace biological markers.
By leveraging richer datasets, dual spectroscopy supports the development of more reliable predictive models and decision-support tools. These improvements ultimately reduce the risk of misinterpretation, support early anomaly detection, and increase confidence in high-stakes applications such as cancer screening, pharmaceutical validation, and petrochemical quality assurance.
5. Flexibility and Durability of Combi Probes
Combi Probes are engineered for deployment in environments where conventional analytical tools are impractical or prone to failure. Their flexible fiber-optic architecture allows for precise spectroscopic measurements in locations that are physically constrained, hazardous, or otherwise inaccessible. This includes narrow reaction vessels, enclosed pipelines, or live biological systems where rigid instruments cannot be inserted without disruption.
Equally critical is the mechanical and chemical robustness of the probe head. Constructed from high-durability materials such as ZrO₂, stainless steel, or chemically resistant polymers, Combi Probes are built to operate under elevated pressures, aggressive chemical environments, and extreme temperature gradients. Probe interfaces can be sealed and jacketed for sterilizable, corrosion-resistant, or flameproof configurations, making them suitable for clean-in-place (CIP) and sterilize-in-place (SIP) operations in pharmaceutical and food production settings.
In industrial field applications — such as petrochemical cracking units, high-pressure bioreactors, and hot solvent loops — Combi Probes maintain optical performance and
structural integrity over extended deployment cycles. Their resilience eliminates the need for probe retraction or sample transfer, reducing downtime and improving overall process efficiency.
This combination of spatial flexibility and environmental durability enables Combi Probes to serve as reliable, real-time diagnostic tools in sectors where few other instruments can operate continuously and accurately.
6. Data Integration and Analysis
The synergy of multimodal spectroscopic methods does not only depend on the probe design but also on the analysis technique employed to extract useful and accurate information from tested samples. Each spectroscopy technique operates on different principles (reflectance vs fluorescence) and generates various signal levels. While the data generated by the different techniques is rich and diverse, it requires cross-modality calibration to ensure the compatibility of measurements.
Reference materials of known spectral features are used to calibrate the instrument’s response. On the other hand, unity-based normalization and wavelength dependent correction factors are applied to generate comprehensive spectra all while accounting for measurement variations inherent to the individual modalities.
Due to the complex and multidimensional nature of the data collected by devices like Combi Probes, advanced chemometric and multivariate analysis techniques are necessary for precise and robust data interpretation.
Principal Component Analysis (PCA) is commonly used to reduce the dimensionality of complex, high-volume datasets while retaining the most significant variance thus facilitating the detection of subtle changes in sample composition. Meanwhile, Partial Least Squares Discriminant Analysis (PLS-DA) supports supervised classification, especially valuable in noisy or overlapping spectral environments. These tools enable Combi Probes to translate complex signals into interpretable and practical insights.
Furthermore, applying preprocessing methods in clinical studies such as second derivative smoothing or SNV normalization have shown improved model performance reporting more than 98% accuracy. This improvement serves as a testament to the synergy of different spectroscopy techniques.
7. Challenges and Future Directions
One of the main challenges facing the integration of Combi Probes in clinical and industrial settings is the need to reproduce consistent and repeatable measurements. Each spectroscopy technique requires interrogation at the same spatial location and with a similar contact pressure. However, manual placement of the probe gives rise to variability in both position and contact pressure. This potential change in pressure can alter the local concentration of certain analytes leading to variations in recorded spectra and reducing measurement accuracy.
In clinical settings, probe cleaning and sterilization pose additional difficulties. To ensure information integrity, the probe needs to be cleaned between different sample measurements and for same-sample measurements as well.
From a hardware perspective, efficient light delivery and collection through a compact and light probe remains a design challenge. As each modality may necessitate its own source, this increases the number of fibers within the probe. This makes system miniaturization especially difficult due to size constraints and risks of deteriorating coupling efficiencies and signal integrity.
The lack of spectral overlap, for instance between fluorescence and mid-infrared modalities, requires the use of two separate spectrometers to detect light at the wavelengths of interest. This complicates integration and increases the cost of the system and its volume.
Finally, while preliminary studies have demonstrated the role of multimodal spectroscopy particularly in cancer diagnosis, larger trials are needed. More comprehensive datasets will allow for the validation of the multivariate discrimination models. These models are crucial for accurate real-time data interpretation and decision-making.
8. Conclusions
Combi Probes represent a new advancement in the field of fiber optic spectroscopy offering real-time, continuous, and remote measurements in a single compact system. The multimodal approach provides a thorough and complete picture of the unit under test, providing details on structural variability, concentration, and chemical composition.
The flexibility and compactness of the design allow Combi Probes to operate in hard-to-reach environments. They can withstand temperature and pressure variations and can be controlled remotely.
Continuous efforts are being made to further develop these new tools, expand their capabilities, miniaturize them, and push for their integration in both industrial and clinical settings.
9. References
- Bogomolov A, Belikova V, Zabarylo UJ, Bibikova O, Usenov I, Sakharova T, Krause H, Minet O, Feliksberger E, Artyushenko V. Synergy Effect of Combining Fluorescence and Mid Infrared Fiber Spectroscopy for Kidney Tumor Diagnostics. Sensors (Basel). 2017 Nov 5;17(11):2548. doi: 10.3390/s17112548. PMID: 29113084; PMCID: PMC5713099.
- Ehlen L, Zabarylo UJ, Speichinger F, Bogomolov A, Belikova V, Bibikova O, Artyushenko V, Minet O, Beyer K, Kreis ME, Kamphues C. Synergy of Fluorescence and Near-Infrared Spectroscopy in Detection of Colorectal Cancer. J Surg Res. 2019 Oct;242:349-356. doi: 10.1016/j.jss.2019.05.011. Epub 2019 May 24. PMID: 31132626.
- Hocotz T, Bibikova O, Belikova V, Bogomolov A, Usenov I, Pieszczek L, Sakharova T, Minet O, Feliksberger E, Artyushenko V, Rau B, Zabarylo U. Synergy Effect of Combined Near and Mid-Infrared Fibre Spectroscopy for Diagnostics of Abdominal Cancer. Sensors (Basel). 2020 Nov 23;20(22):6706. doi: 10.3390/s20226706. PMID: 33238646; PMCID: PMC7700420.
- Novikov A, Perevoschikov S, Usenov I, Sakharova T, Artyushenko V, Bogomolov A. Multimodal fiber probe for simultaneous mid-infrared and Raman spectroscopy. Sci Rep. 2024 Mar 28;14(1):7430. doi: 10.1038/s41598-024-57539-4. PMID: 38548800; PMCID: PMC10978856.
- Bogomolov A, Sakharova T, Usenov I, Mizaikoff C, Belikova V, Perevoschikov S, Artyushenko V, Bibikova O. Fiber Probe for Simultaneous Mid-Infrared and Fluorescence Spectroscopic Analysis. Anal Chem. 2021 Apr 20;93(15):6013-6018. doi: 10.1021/acs.analchem.1c00080. Epub 2021 Apr 6. PMID: 33821623.
- Mitsutake, H., Neves, M. D. G., Rutledge, D. N., Poppi, R. J., & Breitkreitz, M. C. (2020). Extraction of information about structural changes in a semisolid pharmaceutical formulation from near-infrared and Raman images by multivariate curve resolution–alternating least squares and ComDim. Journal of Chemometrics, 34, 1-16. Article e3288. https://doi.org/10.1002/cem.3288
- Andreas Knebl, Di Yan, Jürgen Popp, Torsten Frosch, Fiber enhanced Raman gas spectroscopy, TrAC Trends in Analytical Chemistry, Volume 103, 2018, Pages 230-238, ISSN 0165-9936, https://doi.org/10.1016/j.trac.2017.12.001.